The hand is a crucial part of the body, and the desire to replace it following an amputation – with trauma or cancer being the main underlying causes – is a natural one. Existing bionic hands are already good substitutes for the natural limb yet a number of limitations prevail. These artificial limbs often present low functionality and poor aesthetic appearance and controllability, which deter many amputees from using their prosthesis. This article surveys both the progress made in the field, and the challenges that remain to be overcome.
The need or desire for the functional replacement of a missing upper limb is an ancient one: historically humans have replaced a missing limb with prosthesis for cosmetic, vocational, or personal autonomy reasons. The hand is a powerful tool and its loss causes severe physical and often mental debilitation. Together with the obvious inability to grasp and manipulate objects, an amputee loses the capability to sense and explore the surrounding world as well as the ability to use gestures to support speech and express emotions. Moreover the person may develop psychological problems and encumbrance due to physical differences compared with other people.
Nowadays prostheses are of three types, explained in more detail below: (i) myoelectric, (ii) body powered and (iii) cosmetic. The main prosthetic centre in Italy (INAIL Prosthetic Centre) fits about 100 myoelectric prostheses every year. In Sweden the incidence of upper limb amputees is 50 per year. The number in the UK is about 270 per year (UK Prosthetics Services 2006). If these data are extrapolated to Europe the total number of traumatic upper limb amputees would be 1900 persons a year and the total European community would have approximately 94 000 upper limb amputees.
The most frequent causes of upper limb amputation are trauma and cancer, followed by vascular complications of disease. Transradial level amputations (below elbow) account for 57% and transhumeral (above elbow) for 23% of all arm amputations, with the right arm being more frequently involved in work-related injuries. Upper limb amputations can also be caused by diabetes or other diseases, especially in elderly people. Finally, amputees are one of the most graphic reminders of the destructiveness of war. Thus the need for a versatile prosthetic limb with intuitive motor control and realistic sensory feedback is huge, and its development is a must for the near future.
The need for a versatile prosthetic limb with intuitive motor control and realistic sensory feedback is huge and its development is a must for the near future
Robotic research has been transferred to industry and more functional prostheses have been recently introduced in the market (Touch Bionics, UK). Nevertheless, surveys on using such artificial hands reveal that 30% to 50% amputees do not use their prosthetic hand regularly, typically due to its low functionality, poor cosmetic appearance, and low controllability. It is therefore critical to develop prosthetics that improve the voluntarily-controlled dexterity to allow amputee to perform tasks that are necessary for activities of daily living (ADLs), but that cannot yet be done with today’s state-of-the-art artificial limbs.
Myoelectric Prostheses
During the past years, hand prostheses for amputees have been mainly controlled by processing electromyographic (EMG) signals acquired by means of non-invasive surface electrodes. Now solutions are moving towards a ‘pattern recognition approach’ : (i) signal acquisition from the brain and pre-processing; (ii) feature extraction; (iii) classification; (iv) online/online control that feeds back to improve future pattern recognition. By activating specific muscles not necessarily related to the desired task, the user can select different predefined grasping patterns. Several feature-extraction techniques and pattern-recognition algorithms have been developed to achieve high classification performances.
This approach offers advantages through its robustness, simplicity to implement, and non-invasiveness. Nevertheless it also has several limitations. In fact, the EMG-based control is complicated by the need for coding the different actions of the artificial hand. For example, it is often necessary to control the extension of the fingers of the prosthesis by using other muscles of the upper arm or forearm. In general, it is not possible to use the homologous muscles to control the movements of the prosthetic device leading to the need for a complex algorithm exploiting the potentials of advanced pattern recognition techniques. This is a significant problem for the user, who is not able control the device in a natural way.
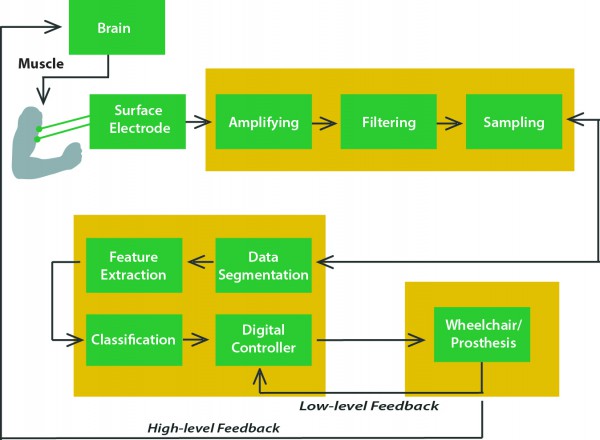
The most important limitation of myoelectric prostheses is the difficulty of delivering any sensory feedback to the user
The most important limitation of myoelectric prostheses is the difficulty of delivering any sensory feedback to the user. These limitations are becoming even more important with the development of more advanced prosthetic arms with built-in sensors. For this reason, in the recent past, some groups have tried to develop alternative methods to restore some similarity with the natural control scheme, e.g. by implanting sensors in the muscles of the forearm or by transferring residual nerves of amputees to other muscles in or near the residual limb. These approaches are potentially very interesting and can provide very remarkable results, but some limits both for the control of the hand and for the delivery of sensory feedback remain.
Another interesting approach could be based on the use of neural interfaces able to create a direct connection with the peripheral nervous system. Despite being challenging this would restore the previously existing connection between the nervous system and the artificial hand and deliver both control and sensory feedback.
Neural Interfaces
The neural interface electrode has long been the limiting technological component for achieving a successful interface to the nervous system. The adequate neural interface should be able to create a selective contact with different fascicles in the nerves in order to restore neural pathways in an effective way. Within the current state of the art, implanted interfaces for the peripheral nerve are divided into main two types: extraneural, implanted around the nerve trunk, and intraneural, which penetrate the nerve trunk.
Extraneural cuff electrodes are reliable and robust and imply a reduced invasiveness, but suffer from a limited selectivity and capability of recording neural signals. With cuff electrodes it is possible to detect the general activity of the nerve, and they have been used to switch on or off the contraction of muscle groups. To improve selectivity, intraneural electrodes (USEA, TIME), inserted into the peripheral nerve, have been developed and tested. This approach seems more promising because it combines acceptable invasiveness with good selectivity.
For selective stimulation of peripheral nerves in the human arm, longitudinal intrafascicular electrodes (LIFEs) have been applied in recent years. The thin-film LIFE (tf-LIFE) marked the development of a functional multi-channel micro-fabricated LIFE structure. This type of microelectrode consists of a polyimide loop with multiple electrode contacts. By use of a second loop with an attached tungsten needle, the tf-LIFE can be placed inside a peripheral nerve and be longitudinally drawn through an individual fascicle to achieve a very close and selective contact. The second loop with the needle is removed after implantation, and the electrode is fixed to the nerve by a suture.
The tf-LIFE has already been applied in one human trial with a bidirectional hand prosthesis. In these experiments the microelectrodes have proved to be suitable for long-term stimulation and recording in the human peripheral nervous system. In particular, the possibility of delivering sensory feedback and decoding different grasping tasks has been shown. In particular, it was shown that pressure sensations can be restored in the patients (confirming what previously achieved by Horch and colleagues), and that it was possible to modulate this feeling by changing the stimulation parameters. Moreover, by processing the neural signals recorded, it was possible to show that it is possible to decode grasping related information which can be used for the online control of the prosthesis.
In the next few months a new clinical trial will be carried out as part of the Italian Ministry of Health’s NEMESIS project, under the clinical supervision of Prof. Paolo M. Rossini (Sacred Heart University in Rome). This new trial carries this research a step further by connecting the prosthetic hand directly to the patient for the first real-time, bidirectional control using peripheral neural signals. Researchers hope to achieve further improvement in the sensory feedback and overall control of the prosthetics with this new method.