Relative to traditional fossil fuels, and even fission, nuclear fusion has long promised - and failed - to safely deliver energy. Yet recent developments provide hope that this elusive technology might finally be ready to emerge as both a viable and inexhaustible energy source in the latter part of the 21st century. Beyond supporting progress and prosperity in the near term, fusion could eventually enable mankind to become a space-faring civilisation, and colonise other planets in the longer term.
The key challenge for scientists, known as 'confinement', is holding the necessary elements together for long enough for fusion to occur. Can recent developments in Inertial Confinement, which uses a decelerating shell of material to try to hold the fusion fuel together for long enough to successfully produce energy, solve this age-old conundrum?
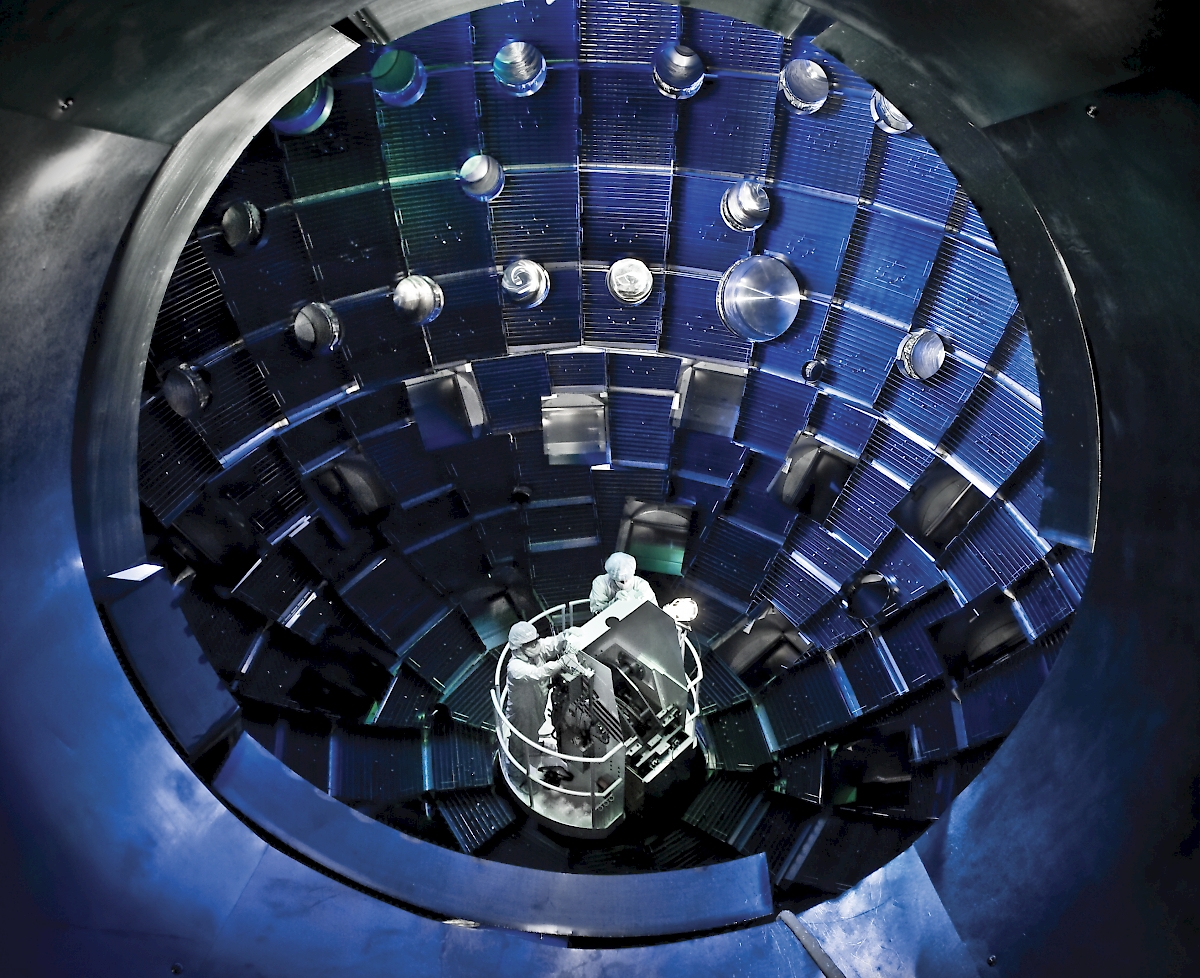
Fusion is the joining of light elements, usually isotopes of Hydrogen, Deuterium (D) and Tritium (T), to produce heavier elements, which releases energy. While a normal Hydrogen nucleus is a single proton, Deuterium is formed when a neutron is added to this and is present as just under 1/6000th of Hydrogen in nature. Fusion occurs when Tritium is added to Hydrogen and Deuterium.
This process, which powers the Sun and other stars, is very much distinct from burning material. It was discovered when, in the mid 19th Century, it was realised that the Sun would only last about 5000 years if made of burning coal - rather than the millions (or, as is now known, billions) of years of existence recorded by geologic records and enabled by its use of fusion power. Fusion can provide the power of the Sun from the same mass for much longer because the energy density in the fuel is so much larger. Boil a kettle and you use the energy contained in 30 grams of coal, however you can boil the water in 12 Olympic sized swimming pools using the energy in 30 grams of the mixture of Deuterium and Tritium (DT) used for fusion. This demonstrates the entirely different energy scale – nuclear rather than chemical – on which fusion occurs, and is in many ways the obstacle to achieving controlled fusion here on Earth.
Everyone is familiar with lighting a fire: a match can be ignited by friction provided by hand which is then used to get kindling to burn to ignite the fire. Energy is successively amplified until we have self-sustaining burn, where the heat of the existing fire heats new areas of coal or wood to a temperature where they ignite. Importantly, at this point the fire takes less energy to heat up a bit of fuel than it then emits when it burns. Fusion tries to utilise a very similar process, where the process of heating up a new piece of fuel which then burns is described as 'thermonuclear burn'.
The term 'thermonuclear' may well be familiar from thermonuclear weapons - this is because that is the only time we have managed to provide a powerful enough match to ignite the fusion fuel is by using a fission bomb to compress and heat the fuel. This is the challenge – to provide such a large match in such extreme conditions to get the fusion reaction going, but without using a nuclear weapon to do it.
Keeping it together
It is this holding together of the kindling while providing the spark which proves to be the key problem due to the high temperatures required to initiate the fusion reaction. The particles in the DT mixture must be moving fast enough to overcome the repulsion between them caused by replusion of positively charged nuclei. The high speed of the particles also manifests itself as a high pressure in the material forcing it apart. The temperatures required are some 100 million Centigrade and therefore the pressures are larger by a factor of about a million than the pressures involved in lighting a fire – it is like trying to light a fire with a hand grenade, you end up with a room covered in coal and kindling, not a nice, self-propagating fire.
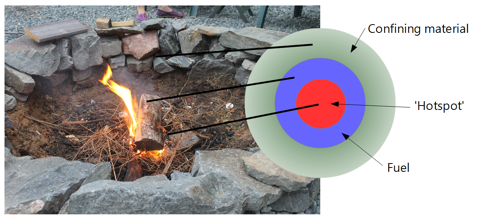
The problem of holding together the kindling for long enough against this immense pressure is referred to as 'confinement', and can be achieved in several ways.
In the Sun it is achieved by gravitational confinement – the material in the Sun is attracted towards its centre by the force of gravity and this holds the Sun together against the pressure of its immensely hot interior where fusion is occurring. This is obviously not practical on Earth.
A second method uses a magnetic field in order to confine a low density plasma in a donut-shaped vessel called a 'tokamak'. Since a charge moving in a magnetic field experiences a force at right angles to both the field and its motion, the ions and electrons in the plasma have to follow the field lines around the torus and so are confined. However, this is not a perfect solution, since collisions and electromagnetic instabilities within the plasma can break this confinement. Considerable success has been achieved with relatively small tokamaks such as JET and MAST at Culham in Oxfordshire, England, and it is hoped that larger tokamaks such as ITER, which is a global project being built in Cadarache in Southern France and which has been the subject of considerable media attention, will achieve further steps on the road to self-sustaining fusion. As of 2016 however, the reactor is still under construction and the current problems are not those of physics but rather those of running a global research project with a multi-billion Euro budget.
Confinement is also achieved in nuclear weapons. As early as the 1950s scientists started to wonder if the methods used there could be adapted and miniaturised to provide a power source. From those ideas Inertial Confinement Fusion (ICF) was born. It works by accelerating a shell of material inwards at high velocities, and then using the force which the material inside that shell must provide to slow the acceleration to confine the fusion process in the centre. Scientific tests performed as ride-alongs to weapons tests, the Halite/Centurion series in the early 1980s, used smaller quantities of fuel and [put] "to rest fundamental questions about the basic feasibility of achieving high gain"1, further adding to the credibility of the ICF concept. In more recent experiments at the National Ignition Facility in California2, researchers are trying to achieve ignition using this method by using short laser pulse fired into a small cylinder called a “hohlraum” to produce an intense radiation field which compresses a capsule at the centre.
Tackling instabilities
While these schemes sound promising, something is still going wrong.
All of the schemes are susceptible to instability in some form. A pendulum hanging downwards under gravity is an example of something in a stable position – it will remain there indefinitely and if slightly disturbed it will swing a bit but then return to its original position. However it is very difficult to balance a pendulum the other way up, or balance a pencil on its point. In this case the system is unstable, hard to set up and any disturbance will not decay but will lead to a complete change in the system. Here, the pencil will fall over to reach a new stable position lying on the table.
In ICF the instability derives from the immense forces which are required and is called the 'Rayleigh-Taylor' instability, which distorts the capsule and mixes its shell with the fuel preventing thermonuclear burn from occurring. Luckily there are ways to mitigate the growth of this instability. The most exciting development in recent years has been the move to the 'high foot design' in late 20133. This uses a difference in the rate at which the hohlraum is heated up to smooth out the interfaces in the capsule and to increase the temperature in the shell as it implodes, both of which act to decrease the rate of growth of the instability.
Employing another approach, at Sandia National Lab outside Albuquerque, New Mexico, compression is being provided not using a laser but by using a machine called a Z-pinch4 which uses an electrical current to generate a magnetic field to compress a cylindrical shell containing the fuel. The set up, called 'MagLIF', is still susceptible to Rayleigh-Taylor instabilities, but the larger energy the Z-pinch can provide means a thicker less breakable shell can be accelerated. It is hoped that the magnetic field can be used to both mitigate instabilities and make it easier for the burn to propagate.
Why should we bother?
Given the immense amount of time, money and effort already expended, and the large future commitment required, to allow us to harness fusion on Earth, it is useful to remind ourselves why fusion is needed.
In the immediate future, the justifications for the importance of fusion are the need for a powerful and inexhaustible energy source. Fossil fuels will be exhausted in the next hundred or so years – the precise timescale is debatable and will clearly be affected by energy availability, population growth and market forces. Renewables – solar, wind or tidal – can provide a stopgap but have fundamental problems in meeting the world's energy budget. Considering solar power in the UK - the amount of power incident on the UK per metre squared is about 100W, and the total area of the UK is just under 250 billion metres squared. Therefore even with 100% efficient solar cells we would have to cover about 1% of the UK with them to produce the 8 x 10^18 Joules per year we use. If you take into account current efficiencies of sub-50%, and the constraints on installing such panels in an economically viable way, this must rise to the 2-3% level. This is not ideal and is similar to the area currently built on – 2.7%, or equivalently somewhere around the area of Kent and Sussex combined. This is further compounded by problems such as power reliability, for example at night, or in the case of wind power on still days, and crucially energy storage.
Fusion power does not currently work, but when it does it would have the ability to provide power as a direct replacement for current fossil fuel based power stations - without the emission of harmful gases and using a fuel which is virtually inexhaustible. Compared to fission power – a normal nuclear power station – the advantage comes from the plentifulness of the fuel, the lack of possibility of a melt-down and the lack of radioactive waste. Decommissioning of fusion power plants would still, however, be expected to be an issue due to the neutron flux through the construction materials.
Despite the immense potential of fusion power, less than £50 million a year in the UK is spent on research on all fusion technologies. Unfortunately, large amounts of money are involved in creating the conditions needed for fusion to occur – NIF for example cost $6 billion – and therefore experiments on fusion energy are difficult to perform in the current funding environment. Taking the internal combustion engine as an example, it is far easier to achieve the goal in a relatively unsophisticated way, as Benz did in 1885 with his Patent-Motorwagen capable of about 10 mph, and then improve incrementally on that design to obtain the modern automobile. Funding and time constraints mean that researchers in fusion do not have this luxury of designing away from a working model making it more efficient and cost effective, but rather must solve a problem equivalent (in this analogy) to designing a modern car from scratch but using a budget of one fortieth of the £2.1 billion spent by Mercedes-Benz, one of many car manufacturers, on research and development every year. Given the comparative underfunding of fusion, it is hardly surprising that it is constantly 50 years in the future.The lack of funding also leads to a drain of researchers from the field; while places on PhD programmes are, relatively, plentiful, the rate of moving those scientists onto post-doctoral positions, into fellowships or into permanent jobs in the UK is very low. Only a few percent of people completing a PhD in fusion research enter a permanent academic job in the UK, a situation which it must be emphasised is common to academic physics research in general. These researchers are not being lost because of a lack of ability: they are highly sought after, and employed, by foreign laboratories, universities and multinational companies.
To boldly go...
Measures of quality of life such as access to water, life expectancy, and years of schooling5 are all correlated with an increased rate of energy consumption per capita. It is of course hard to show causation rather than correlation, but it would seem that making low cost, politically unencumbered energy available to the world's poorest countries and people would be advantageous. However while the usual justifications for the importance of fusion - its ability to replace fossil fuels, and remove their harmful environmental impacts, to reduce competition for resources or lift people out of energy poverty - are compelling in the near term, to see the real reason why fusion is worthwhile we should look to the recent 'Star Wars' or 'Star Trek' films.
There we see large starships flying effortlessly between planets and stars, clearly far beyond current technology. Say that you wish to accelerate a spaceship to high speeds using a fuel it carries on board and let us pretend the half the ship could be made of fuel, then how fast could it go? Using a fossil fuel based ship the maximum speed is a mere 18,000 mph; using fusion, speeds of about 100 million mph, 15% of the speed of light, could be reached. Mars in an hour and a half rather than years, the nearest star in 30 years rather than in excess of 100,000. This is the real reason for achieving fusion – the ability to provide a ready, inexhaustible source of energy to allow us to reach beyond the Earth to colonise nearby planets or stars. This will enable the human race to become an advanced, space-faring civilisation - potentially ensuring the long-term survival of the species - and that is surely a worthwhile goal.